Fluorescence as a tool in microscopy
Fluorescence is widely used in microscopy and an important tool for observing the distribution of specific molecules. Most molecules in cells do not fluoresce. Therefore, they have to be marked with fluorescing molecules called fluorochromes or fluorophores. The molecules of interest can be labeled directly, e.g., DNA with DAPI, or they can be immunostained with fluorochromes that are coupled to specific antibodies. Fixation of the cells is usually necessary for immunostaining.
Fluorescence microscopy also allows time lapse imaging of living cells or tissue. For this purpose, proteins of interest can be tagged with genetically encoded fluorescing molecules like GFP (green fluorescent protein). Molecules of interest,e.g., Ca2+, can also be tagged using reversibly binding synthetic dyes, e.g., fura-2, or genetically modified naturally occurring proteins, e.g., GFP-derivatives.
Changes in the energy states of electrons lead to luminescence
Luminescence describes the occurrence of luminous effects that are caused by the change of an electron within a molecule or atom from an excited state with higher energy to another state with lower energy [2]. Electrons can exist in different energy states. The ground state is a very stable state for an electron and has the lowest energy level. If electrons absorb energy, they can be elevated to a higher energy level, an excited state. As the excited state is of higher energy than the ground state, energy has to be released when an electron returns to its ground state. This energy can be released in the form of emitted photons.
There are several forms of luminescence differing in the way the system is excited. Electroluminescence is where the system is excited via an electric current, chemiluminescence occurs due to a chemical reaction, and photoluminescence results from the excitation via photons .
Photoluminescence can further be divided into two sub-groups: fluorescence and phosphorescence [2]. The main difference between fluorescence and phosphorescence is the duration of their luminescence. Fluorescence immediately ends when illumination is stopped. In contrast, phosphorescence can last for minutes or even hours after the excitation has ended.
The mechanism of fluorescence
Fluorochromes will only fluoresce if they are illuminated with light of the corresponding wavelength. The wavelength depends on the absorption spectrum of the fluorophore and it has to be ensured that an appropriate quantity of energy is delivered to elevate the electrons to the excited state. After the electrons are excited they can dwell in this high energy state for a very short time only [4], typically on the order of nanoseconds. When the electrons relax to their ground state or another state with a lower energy level, energy is released as a photon. As some of the energy is lost during this process, light with an increased wavelength and lower energy is emitted by the fluorochrome compared to the absorbed light.
The mechanism of phosphorescence
As phosphorescing molecules can luminesce for a much longer time than fluorochromes, there must be a difference in the way they store the excitation energy. The basis for this discrepancy is found in the two forms of excitation levels, the singlet excited state and triplet excited state which are based on different spin alignments.
Spins are an attribute of electrons. In simplified terms, the spin describes the angular momentum of the electron caused by its rotation. The orientation of an electron’s spin can be positive (+1/2) or negative (–1/2). Spin pairs of higher energy levels can either be parallel or antiparallel in their orientation to each other. In antiparallel spin pairs the individual angular momentums compensate each other and the total spin gets a value of zero. This spin alignment is called a singlet state. Two parallel spins do not compensate and get a value different from zero. In this case the spins are said to be in a triplet state.
Fluorescence occurs when electrons go back from a singlet excited state to the ground state. But in some molecules the spins of the excited electrons can be switched to a triplet state due to a process called intersystem crossing (ICS). ICS is influenced by spin-orbit coupling which involves the interaction of the magnetic moment of an electron due to its spin and orbital angular momentum. These electrons lose energy to their surroundings until they are in the triplet ground state. This state is of higher energy than the ground state, but also of lower energy than the singlet excited state. Therefore, the electrons cannot switch back to the singlet state, nor can they easily go back to the ground state, as only total spins with a value of zero are allowed due to quantum mechanics. The molecules are therefore trapped in their energy state.
But a few changes from the triplet ground state to the ground state are possible at one time. These changes during intersystem crossing give rise to the emission of photons and phosphorescence. As only a few events are possible at one time, the triplet ground state presents a kind of energy reservoir, making phosphorescence possible over a longer time period. Phosphorescence, therefore, describes photon emission during the transition of an electron from a triplet state to the ground state.
Luminescence in microscopy
For microscopy, fluorescence is the most useful kind of luminescence. Fluorochromes can easily be excited with their specific wavelength via certain light sources, e.g., lamps and filter systems or lasers, and the emitted light can be distinguished from the excitation light by the wavelength (Stokes’ shift).
Using fluorescence imaging, scientists can characterize the amount and localization of fluorescent molecules inside a cell. Another advantage of fluorescence microscopy is that several fluorochromes can be used simultaneously. The fluorochromes only have to vary in their excitation and emission wavelength. Hence, different target molecules can be observed simultaneously, allowing a large variety of experiments, like co-localization studies, to be performed.
Examples of images recorded with fluorescence microscopy
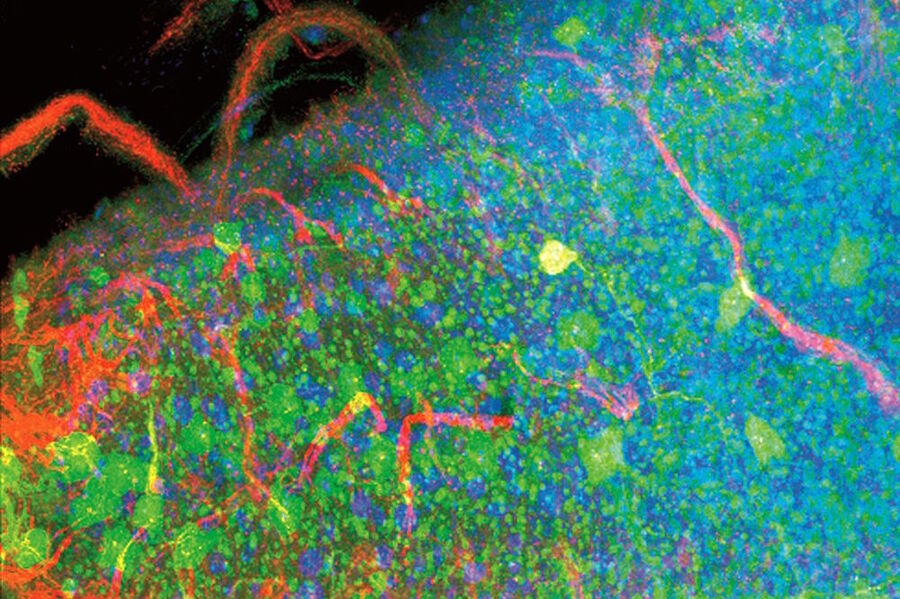
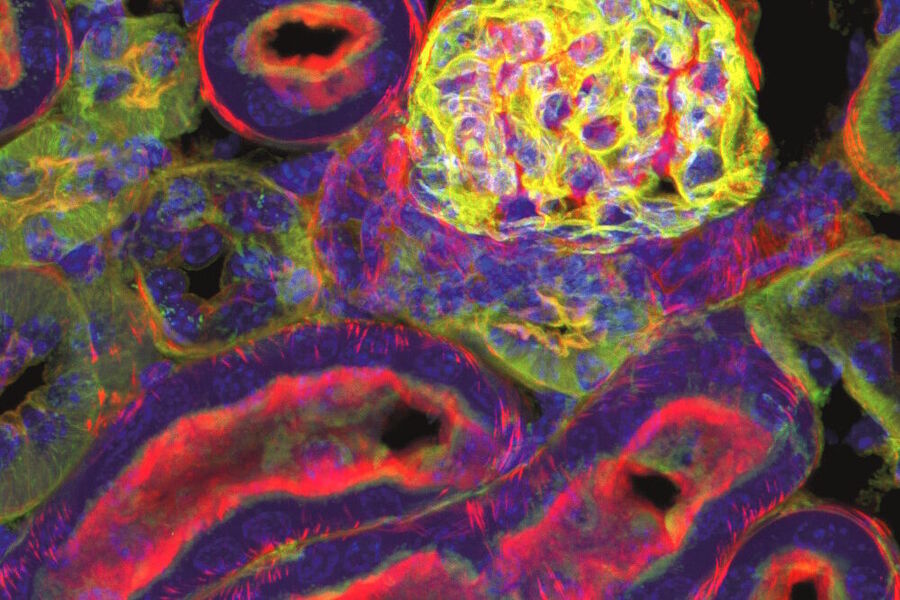
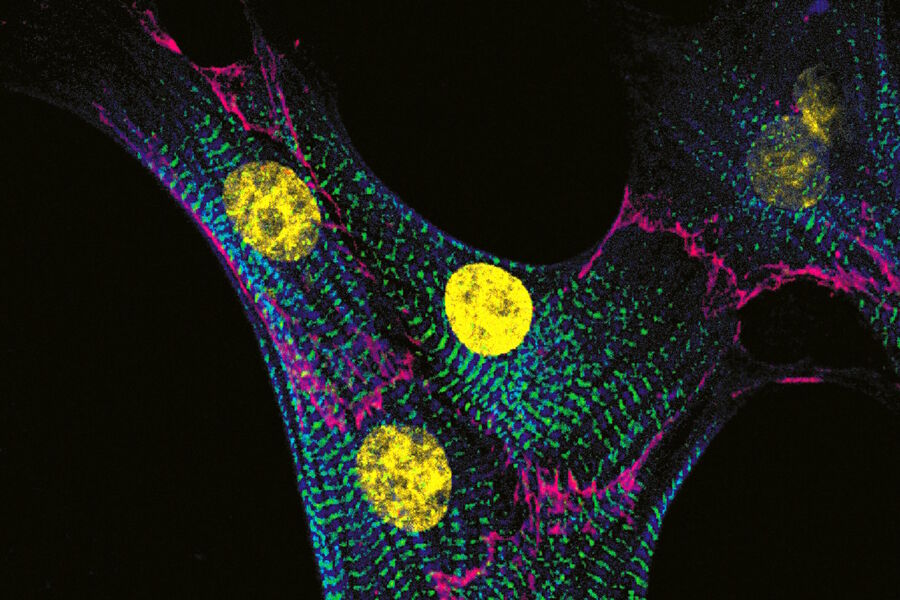
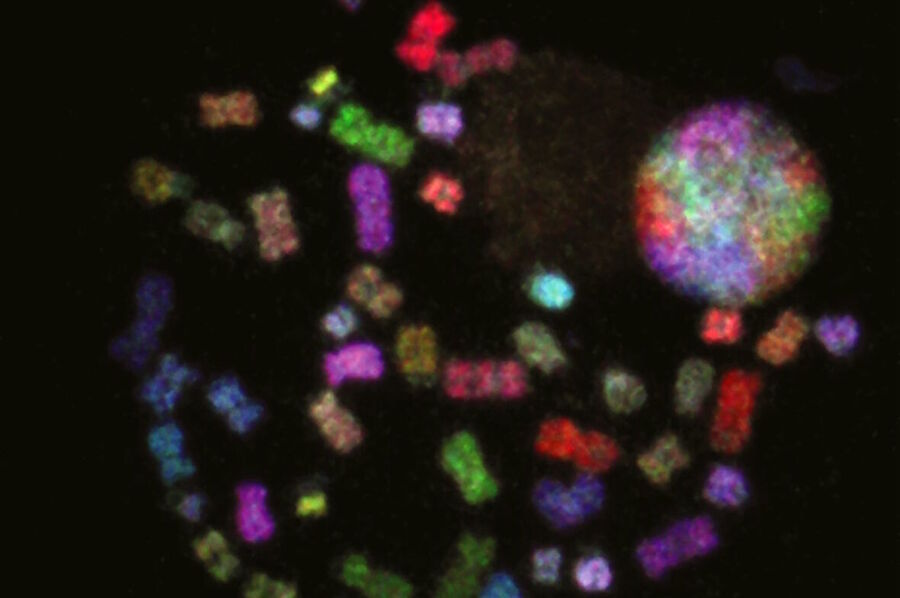