Three wave mixing experiments
The first recordings of Coherent Anti-Stokes Raman Scattering go back to the 6os of the last century, when two researchers of the Scientific Laboratory at the Ford Motor Company, P. D. Maker and R. W. Terhune, published an article about their experiments. They simply called their work "three wave mixing experiments" [1]. Almost ten years later, in the middle of the 70s, R.F. Begley, A.B. Harvey and R. L. Byer of Stanford University showed advantages of CARS over Raman spectroscopy and first applications on biological samples [2]. Since then, the development of CARS has been inexorable. In 1999, A. Zumbusch, G. R. Holton and Xie, X. S. reported about "Vibrational Microscopy Using Coherent Anti-Stokes Raman Scattering" [3], and after Potma’s and Xie’s article written in 2004 "CARS for Biology and Medicine" [4] the Coherent Anti-Stokes Raman scattering technique had arrived in the life science sector.
Meanwhile, the number of publications about CARS increased from just twenty in the year 2004 to more than seventy-five last year, including more and more applications with a biological and medical background.
Physical background of CARS
CARS is a third-order nonlinear process that involves a pump beam at a frequency ωp and a Stokes beam at a frequency of ωs. The signal at the anti-Stokes frequency of ωas= 2ωp- ωs is generated in the phase-matching direction. The sample is stimulated through a wave-mixing process. The vibrational contrast in CARS is created when the frequency difference Δω=ωp- ωs between the pump beam and the Stokes beam is equal to the frequency of the molecular vibration of a particular chemical bond and oscillations of molecules with that bond are driven coherently.
The CARS signal is generated from vibrational motion of the molecules in the sample. No external markers are required to get a CARS image. Different types of molecules show characteristic vibrational energy states. Electromagnetic energy at appropriate (infrared) wavelengths will excite the molecules into these states. To generate sufficient signal above noise, the CARS concept populates a characteristic vibrational state by pumping first from ground state into a virtual state. This is achieved by illumination with a "pump" beam at a medium wavelength (ωP). The energy of the photons must be less than the difference from the ground state to the first excited state. Upon simultaneous illumination by a second beam (Stokes beam) at a longer wavelength (ωS), the molecule is forced from the virtual state into the desired vibrational state. As the pump beam is tunable, the residual difference ωΔ = ωP-ωS can be specifically adjusted to the desired vibrational energy of the relevant molecule. In essence, the desired molecule population is converted into a vibrational state. To visualize the molecules, the pump beam raises the electronic system into a second virtual state of the energy ωP+ωΔ. From here, the molecule is allowed to relax back to the ground state, while the energy ωP+ωΔ = ωAS is detected (Anti-Stokes beam). These photons are used for imaging.
The CARS process in detail
F-CARS and E-CARS
The CARS signal can be detected in two different directions:
1. Forward CARS (F-CARS), detected with transmitted light detectors
With an accurately defined filter set the signal is detected in the phase-matched direction. F-CARS signals are generally very strong and can be differentiated clearly from the often strong non-resonant background (which arises from the surrounding solvent). Forward CARS is applicable to thin samples.
2. Epi CARS (E-CARS)
If a weak F-CARS signal is overshadowed by the non-resonant background, the contrast can be enhanced by detection in the backward direction, which reduces the noise of the image. E-CARS is particularly sensitive to objects in focus that are smaller than the optical wavelength. When the sample is highly scattering, the forward propagating CARS signal can be backscattered, leading to a strong epi signal.
Why CARS for microscopy?
Confocal and multiphoton imaging techniques visualize typical structures or dynamic processes in biological samples and depend on existing autofluorescent matter in the specimen or the availability of suitable fluorescent dyes. As a consequence, new dyes are required to analyze unknown and potentially relevant details in biological samples.
Staining standard fluorescence samples is often time-consuming and expensive and may influence typical properties of living cells as they act in a chemical way. Furthermore, dyes lose intensity and alter the sample. They often cause phototoxicity, harm the specimen and consequently may influence the result of the experiment.
CARS overcomes these drawbacks by the intrinsic characteristics of the method. CARS does not require labeling because it is highly specific to molecular compounds which are based on vibrational contrast and chemical selectivity [1-5]. The crucial advantage of this method is that the sample remains almost unaffected.
With CARS, new samples that could not be stained due to unavailability of the appropriate dye are now accessible.
By integrating CARS technology into a confocal system, the drawbacks of conventional microscope techniques can be overcome. The latest commercial developments result in an easy-to-use and efficient imaging microscope for a variety of biological and non-biological samples. Two infrared laser beams which are adjusted exactly in terms of spatial and temporal properties generate brilliant CARS images at different wave numbers. The combination of a couple of CARS filters and non-descanned detectors allows the detection in the forward as well as in the backward (epi) direction. Recording of the second harmonic generation (SHG) can be done simultaneously. Combinations of conventional and high-speed scanners support the analysis of dynamic processes at video rate as well as morphological studies at high resolution.
Applications – Biology, Food, Cosmetics
Biology
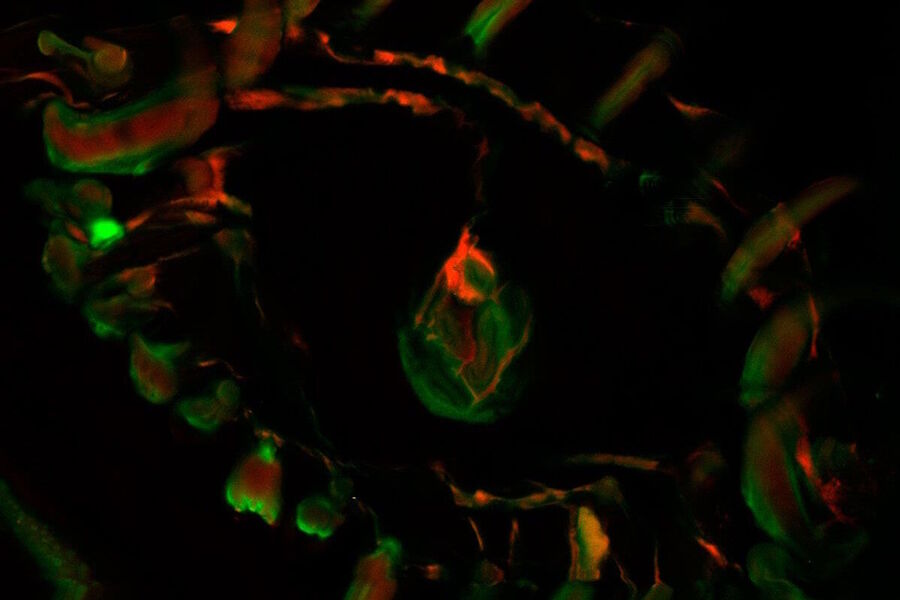